New Unit Profile: Searching for Simplicity
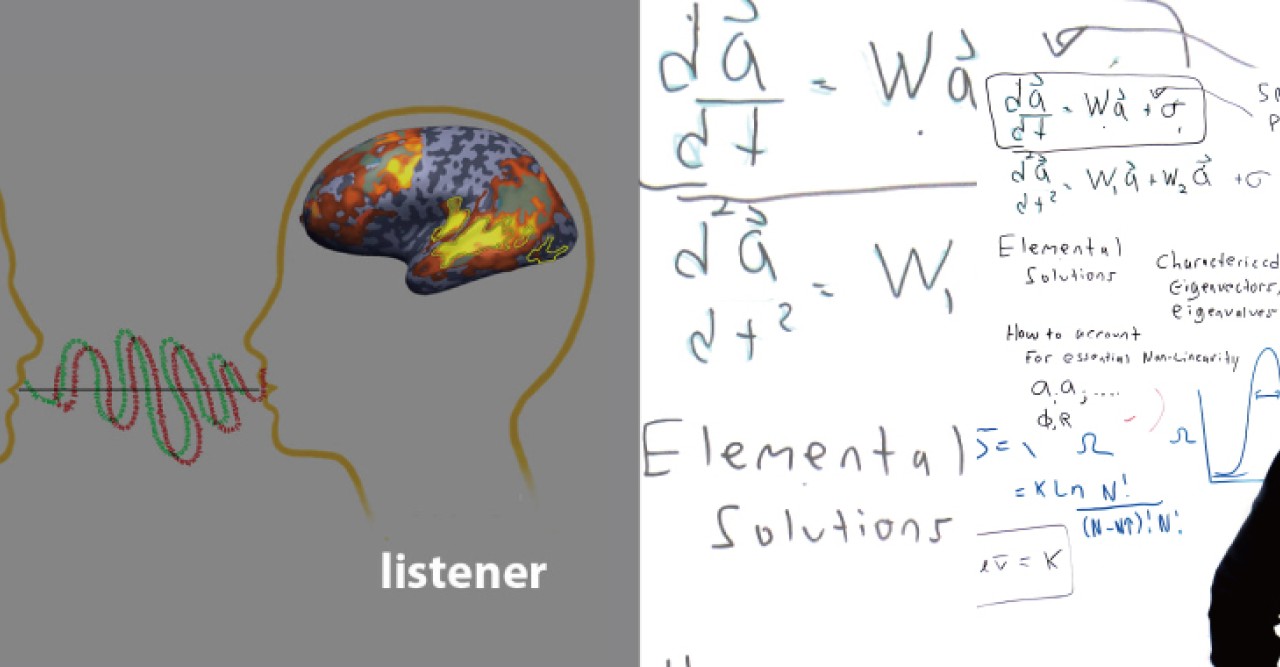
Physicists have long searched for universal laws that explain the nature and properties of matter and energy on scales as large as the universe and as small as a proton, but until relatively recently, complex biological systems, like the structure of DNA and the nature of human thought, have remained uncharted territory.
“In physics we learn that electromagnetism, gravity, and strong and weak nuclear forces are the fundamental interactions of the universe,” says Professor Greg Stephens, head of the new Biological Physics Theory Unit at OIST, “but understanding the brain that we use to uncover these laws certainly seems just as fundamental as the laws themselves.”
Trained in theoretical physics and currently working to understand the living world, there is no doubt Stephens feels at home in OIST’s interdisciplinary environment. “My work is highly collaborative and at OIST the barriers to working across fields are remarkably low,” he says. Stephens also holds an Associate Professor position in the Department of Physics and Astronomy at Vrije Universiteit Amsterdam in the Netherlands, where he also conducts biophysics research.
As a field biophysics gained traction in 1944 when Erwin Schrödinger, a Nobel Laureate and theoretical physicist, published What is Life? – a book based on public lectures that discussed how physics and chemistry might account for living organisms. Revolutionary for its time, the book helped discount the idea that life was outside the scope of physical science. Since then, most of biophysics has focused on molecular phenomena, including the analysis of DNA, RNA and proteins.
At OIST Stephens works on the scale of systems, an endeavor only possible with the advent of new biophysical experiments. “Today we can measure many molecules within the cell, many neurons in a brain or many birds in a flock,” he says. “These data offer a comprehensive picture of the system as a whole and guide our analysis towards finding principles of function.”
While a post-doc at Princeton University, Stephens and colleagues in cognitive science conducted a study that elucidated the relationship between brain activity and human communication. The group used functional magnetic resonance imaging (fMRI) to record a speaker’s brain activity while telling a story and listeners’ brain activity while hearing the said story. The data not only showed that the activity in the listeners’ brain often paralleled that in the speaker’s brain, but also that this parallel corresponded to the listener’s comprehension: the stronger the parallel in the brains of the speaker and listener, the more the listener could recall about the story afterwards.
“Though the human brain is far from a traditional physical system, my background gave me the tools to construct a simple mathematical model that allowed us to assess the extent, location and timing of the similarity between the speaker and listener’s brain activity,” says Stephens.
Stephens also searches for patterns at the other end of the animal kingdom – namely, in the behavior of Caenorhabditis elegans, a transparent worm about one millimeter in length. Interestingly, OIST’s own Professor Sydney Brenner popularized the use of C. elegans as a model organism when he began research on its development and molecular biology in the 1950’s.
“The movement of C. elegans is beautiful to watch – it’s clearly not random, but it’s also not easy to characterize,” says Stephens. “We wanted to find a mathematically precise description of the worm’s motion that was still a good match to the data.”
One approach is to reconstruct all of the neurons, synapses and muscles that make up the worm’s body and then to simulate its behavior. “Even though C. elegans has only a few hundred neurons, there isn’t enough known detail to make such a model,” adds Stephens. “As with many other examples in biology, the behavior of the system is hard to predict from the sum of its parts.”
Instead, Stephens and colleagues at Princeton University directly analyzed high-resolution videos of the worm’s movement and discovered that four prototypical shapes could account for essentially all the wiggles in the worm’s body shape during movement. These prototypical shapes also allow for a unified description of behavior across a diverse range of worm species, which can be as genetically different from each other as we are from fish.
“Physics is more than just the study of matter and energy, it’s an intellectual culture in which simple models and general principles have far-reaching applicability,” adds Stephens. “It’s a wonderful challenge, both to physics and to biology, to explore just how just how far simple ideas can go.”
Specialty
Research Unit
For press enquiries:
Press Inquiry Form