New Unit Profile: From Magic to Quantum Machines
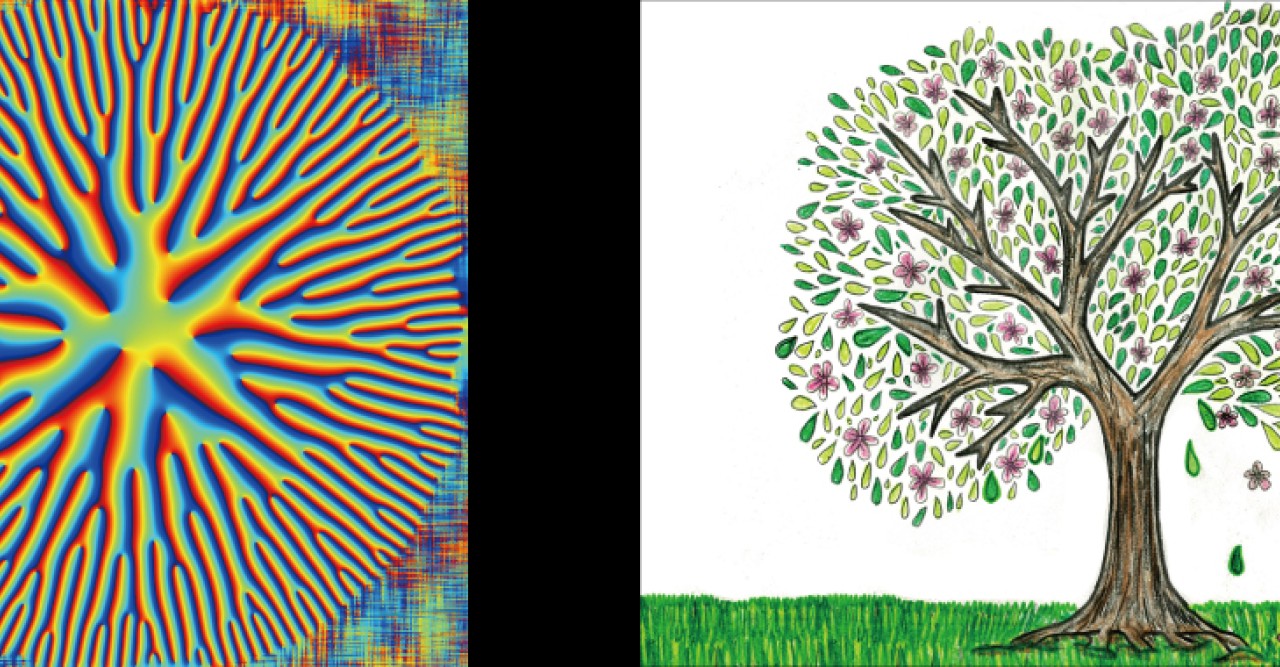
While some languages are more difficult to translate than others, the hardest by far is mathematics. And if there's a dialect of math that boggles more minds than any other, it’s the complex equations associated with quantum physics.
“Quantum theory aims to explain phenomena that occur at temperatures and on scales that humans are too big and too hot to experience," says Professor Thomas Busch, head of the new Quantum Systems Unit at OIST. “We don’t have words for things we don’t experience, so it’s difficult to accurately describe our work with language.”
Though the language barrier may hinder quantum physicists from linguistically expounding their work as precisely as biologists, they will continue to try – quantum theory could fundamentally change the way we understand the world and lead to revolutionary technologies.
Two of the most important, yet strangest, phenomena that occur on a quantum scale are wave-particle duality and superposition. These concepts are best illustrated through the double-slit experiment, first performed by Thomas Young with light during early 1800s and reconfigured with matter in the 1960s by Clauss Jönsson. By shooting an electron at a wall with two slits positioned in front of a screen, Jönsson and latter physicists found that matter exhibits the characteristics of a wave in one case and a particle in another. If the researchers measured the system while it was evolving, each electron went though one slit as a particle, but when the measurement was taken at the end, each electron went through both slits as a wave.
Why does measurement collapse the quantum state of matter? Physicists are still searching for the answer to this question. “To some extent, measurements cause the system to lose its quantum properties just like measuring the pressure in a tire causes the tire to lose air,” explains Busch. “Another part of the battle is coming to terms with the fact that words like ‘particle’ and ‘wave’ are just not sufficient for describing a quantum state appropriately.” While the concept of superposition, or a material object being in two places at once, is difficult to grasp linguistically, it can be easily illustrated with equations. But “these phenomena aren’t just mathematical concepts – they are occurring in the real world,” he adds.
To make the situation more complex, a single electron can be 90 percent going through the left slit and 10 percent the right or 67 percent the left and 33 the right. “All these superpositions are possible,” says Busch. “And the number of combinations is only limited by what’s experimentally possible.”
The Unit’s main aim is to theoretically demystify quantum phenomena, like superposition, that are close to what experimental physicists can produce today. “The laws of quantum mechanics allow for engineering and operating technologies that are fundamentally different from what we have presently,” says Busch. A quantum computer, for example, would be able to harness the nearly infinite possibilities of electrons in superposition, while current computers process bits of information using binary logic: 0 or 1 (i.e. on or off, the left slit or the right slit). A quantum computer bit could be on or off or both at the same time (e.g. 90 percent on and 10 percent off). By expanding the possibilities of what state a bit can be in, quantum bits would be able to process information at speeds considerably faster than those of today.
But there's a catch: Keeping a computer bit in a quantum state is a difficult task – like humans, current bits are too big and too hot to sustain a quantum state. Busch’s group works to identify and describe systems that aren’t too large or hot to find pathways for developing technologies like quantum computers.
Members of the group are also working to understand physical processes that occur in nature, like photosynthesis, on the quantum level. While researchers know that plants use sunlight to produce nutrients from carbon dioxide and water, it’s unclear why chlorophyll, the green pigment in plants, is such an excellent receptor for light. During photosynthesis, chlorophyll molecules absorb one photon and lose one electron, causing a chain reaction that leads to the production of sugar, a plant's sustenance, and the release of oxygen. Could chlorophyll’s efficiency at transferring light energy to chemical energy be due, in part, to the nearly infinite possibilities of quantum interactions? Recently, physicists observed quantum coherence occurring in chlorophyll molecules, a phenomenon where two or more particles of matter take on the form of a wave and combine to act as one large wave – a system researchers in Busch’s Unit are also studying. While it is not clear if these laboratory experiments translate into the real world, it is a promising beginning.
“There are a lot of quantum phenomena that we don’t fully grasp or have proper words to describe, but this should not stop us from making good use of them,” says Busch. “In the words of Arthur C. Clarke, author of 2001: A Space Odyssey among other things, ‘the only way of discovering the limits of the possible is to venture a little way past them into the impossible. Any sufficiently advanced technology is indistinguishable from magic.’”
Specialties
Research Unit
For press enquiries:
Press Inquiry Form